Biomass combustion
The control of fire is considered to be a landmark and a turning point in human history. Biomass burning, initially to provide heat for cooking and protection from predators, is the most important form of renewable energy ever used.
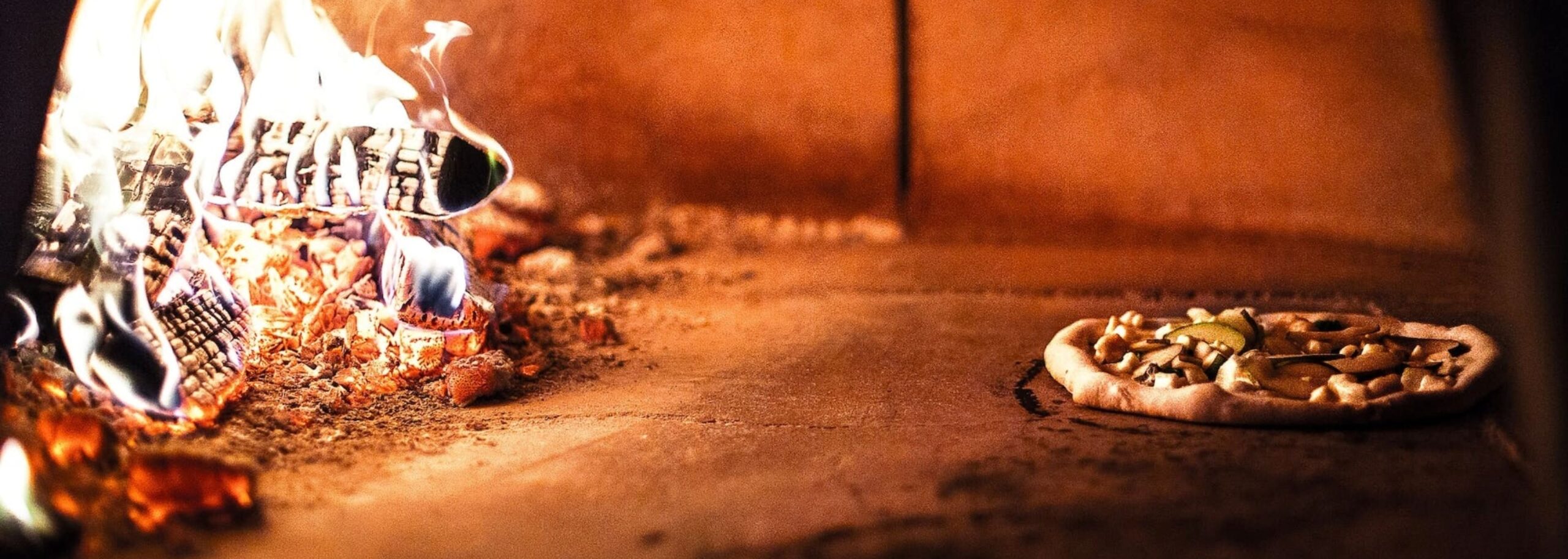
Cooking is one of the oldest uses of bioenergy by humans. Today, modern biomass combustion is providing different forms of sustainable energy in multiple applications (Photo credit: Pexels/Ponyo Sakana)
The development of what we call modern biomass combustion technologies—aiming for higher efficiencies and pollution control—dates back to the 1970s when the oil crises revealed the world’s dependence on fossil fuels. The long tradition of biomass use throughout history and its abundance around the globe were the key factors in the quick deployment of modern biomass combustion.
Modern biomass combustion, which has gone through almost 50 years of development, today offers technologically mature solutions across the whole capacity range, from small- scale residential applications with several kilowatts of electricity output to large power plants and combined heat and power (CHP) plants outputting hundreds of megawatts.
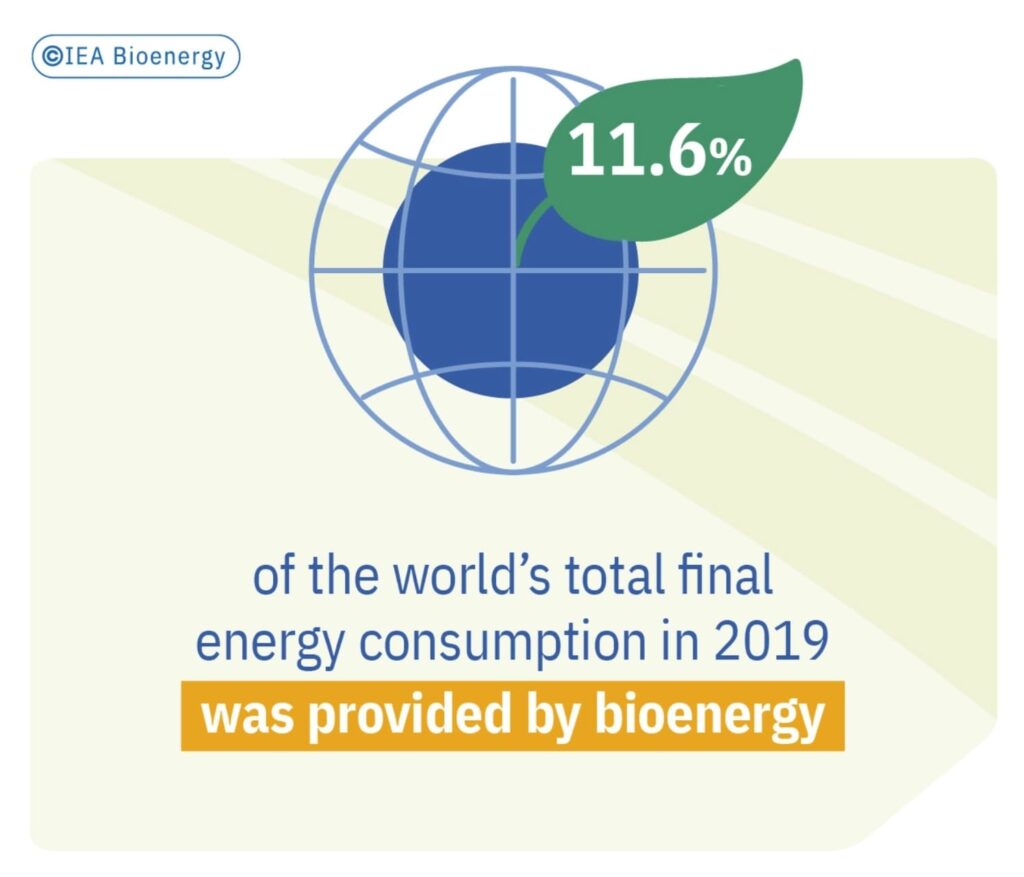
Residential applications
Biomass combustion systems are providing low temperature heat up to 100°C for heating and hot water supply in buildings. Such applications can be used either in individual residence/building heating solutions or in central systems distributing heat to buildings via a heating grid (also referred to as district heating).
Small-scale heating systems
Wood-based fuels dominate the market for small-scale biomass combustion systems. In terms of applications, direct heating systems provide thermal energy to the installation space, while central or hydronic heating systems transfer the energy to a heat transfer fluid, usually water.
Traditionally, firewood is still the most common fuel in this sector, but automated systems based on wood pellets or wood chips are steadily increasing their market shares. This trend results mainly from the significant comfort gain that comes with automated fuel supply, but in some countries, the shift from manual to automated systems is also being supported by policies.
While modern units such as pellet stoves and boilers reach high efficiencies of more than 90%, many systems in service are operated with limited efficiencies and high emissions. Due to products having long lifetimes of 20 years or more, many appliances now in use are no longer state-of-the-art. It is thus necessary to replace old and inefficient units with modern appliances to ensure that more efficient and less polluting bioenergy is produced.
District heating
In areas with high heat-demand densities, the installation of heating networks with central units instead of distributed individual heating systems is often advantageous. District heating systems are very common in several countries such as Austria, Denmark, and Sweden. Larger combustion systems benefit from the economy of scale and the availability of efficient flue gas treatment systems. This usually allows lower quality fuels to be used, such as wood chips from forest residues, with concomitant economic benefits.
A major drawback of district heating systems is the relatively high investment costs for the district heating lines and resulting long payback periods. For existing heating networks fed with fossil heat, the conversion to biomass-based heating is usually an economically attractive option.
The economic case for biomass-based district heating is quite complex, as several parameters influence both the costs and the turnover. High overall system efficiency is one of the main keys to successful district heating projects. Quality management programmes have been developed to support effective planning and operation of district heating networks (e.g., Quality Management system for Biomass District Heating Plants).
The biomass combustion experts from Bioenergy Task 32 of the International Energy Agency (IEA) have recently compiled case studies of innovative biomass-based heating applications in various sectors: Bioenergy for heat – the Hot Cases.
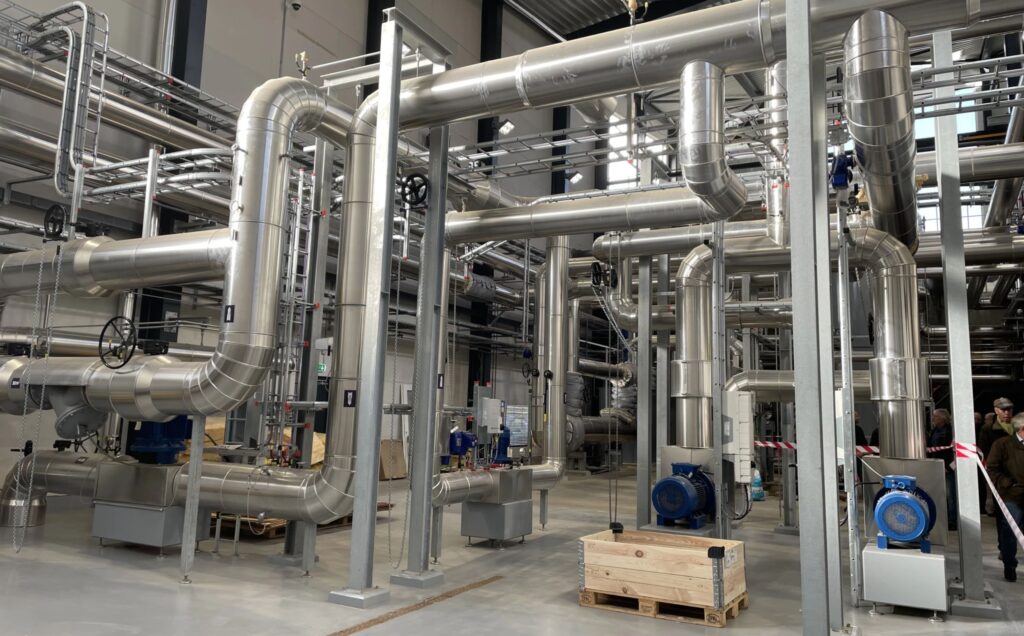
Industrial applications
Process heat
The decarbonisation of industrial heat demand is particularly challenging due to the varying technical requirements of the applications used. Process heat is often required at high temperatures, which reduces the options for renewable heat technologies. Biomass combustion systems can provide high temperature heat up to 500°C via different heat transfer fluids— the most common being steam, thermal oil, and hot gas/air. For even higher temperatures, biomass combustion is applicable if direct heating via the flue gases is an option. This is why decarbonisation of industrial heat demand is seen as a future key market for biomass combustion systems.
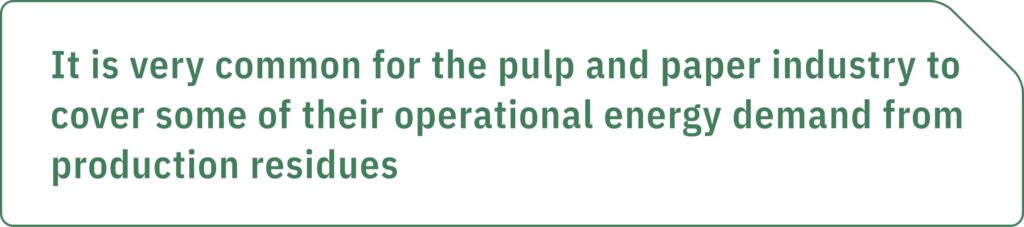
The market penetration of biomass-based process heat applications, however, is often limited to industry sectors with direct links to biogenic material. For instance, it is very common for the pulp and paper industry to cover some of their operational energy demand from production residues (e.g., black liquor). Similar concepts of on-site energetic use of by-products are also frequently applied in the food and feed industry. Good examples were recently published by experts of IEA Bioenergy Task 32:
- Wood chip combustion for process steam in a potato processing industry
- Combustion of wood chips and grain residues for process heat supply in the largest bakery in Switzerland
In contrast, industrial sectors with no relation to biomass have frequently shown limited interest in renewable heat concepts. The main reason is costs. Due to fossil-based energy prices in industry being historically quite low, it is often difficult to find economically competitive biomass-based solutions, unless policy-based market corrections such as carbon pricing or subsidy schemes are in place. Consequently, industry energy prices and regulatory framework conditions can be identified as keys to success for biomass-based process heat applications.
Combined heat and power
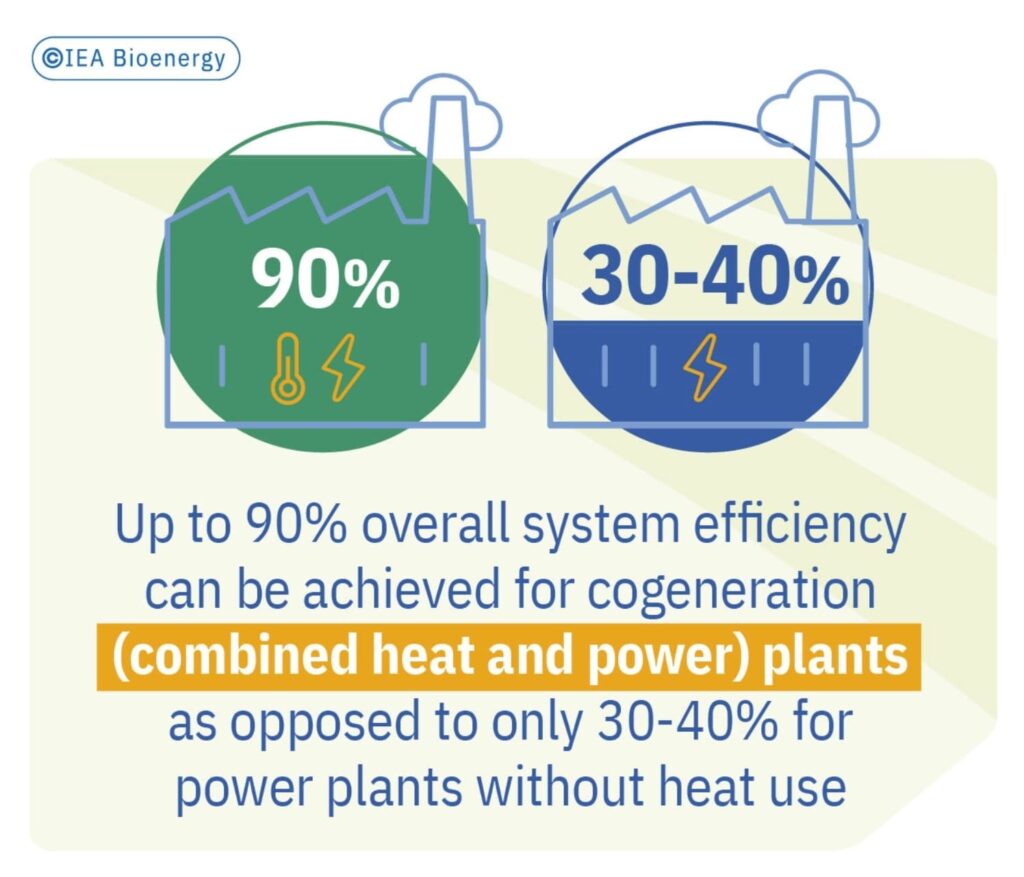
Active flue gas condensation, for example with a heat pump, can increase system efficiency even further. In cases where the heat is mainly used for residential heating, the heat demand in summer will be significantly lower than in winter. In such cases thermal cooling concepts can be considered which use the excess heat during summertime to generate cooling energy via absorption or adsorption heat pumps. Alternatively, biomass CHPs could be operated only in the cold season when there is sufficient heat demand; this practice is already being applied in some regions.
In the low-capacity range, alternative power technologies like Organic Rankine Cycles (ORC), Stirling engines, or thermoelectric generators are applied in addition to steam-based processes. While a few products are already being successfully marketed, several are still in the demonstration phase. A good overview of best practice solutions for decentralised CHP systems and the state of technology of small-and micro-scale CHP technologies has recently been published by IEA Bioenergy Task 32.
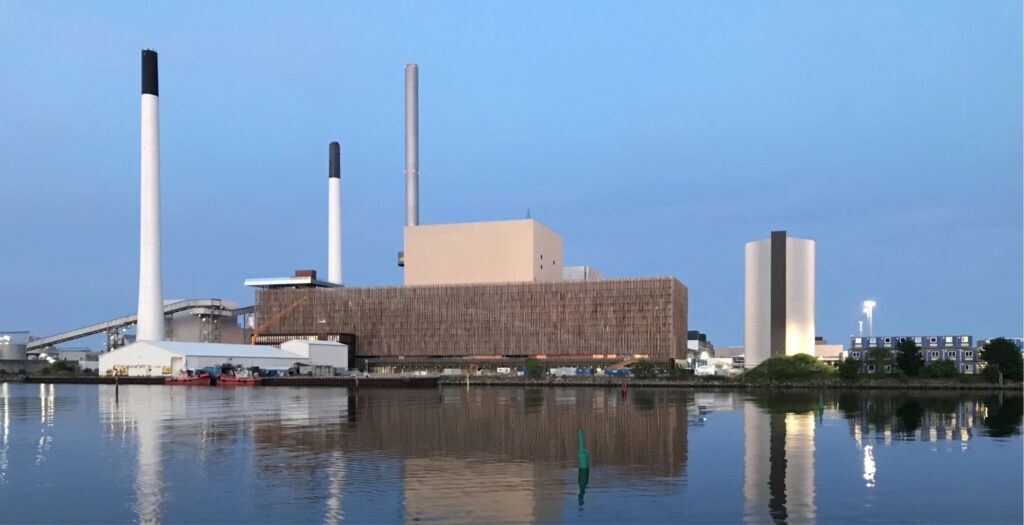
Power plant applications
Rankine steam cycles are still the most common thermal power applications in the world energy system. The heat used to produce the steam can be generated through biomass combustion. Biomass power plants apply different combustion technologies depending mainly on the type and quality of fuel they are using. Grate- and fluidised bed–fired systems are very common, having proven their reliability in many applications.
Erecting dedicated biomass power plants usually requires long-term fuel delivery concepts and contracts. Economies of scale help in reducing the specific costs; nevertheless, biomass power plants require significant investments and relatively long payback times, depending on the regulatory framework conditions in place.
In contrast, the full conversion of existing coal power plants to use biomass feedstocks appears to be promising in terms of reducing high investment costs. However, experience—not least with dust explosions—shows that many modifications, predominantly in the fuel and ash handling and preparation phases, are necessary to ensure the safe and reliable operation of such plants. Pre- treatment options such as steam explosion or torrefaction of the fuel can significantly reduce these efforts and the corresponding costs, as they can modify relevant fuel characteristics like milling properties, water resistance, and dust formation tendencies. Task 32 has studied steam explosion process technology as a part of a project on fuel pre-treatment.
Co-firing biomass in coal power plants
In terms of its potential for cutting greenhouse gas (GHG) emissions, the cofiring of biomass in coal power plants is very attractive. Only minor modifications to the plant are necessary to replace up to 50% of the coal with biomass, if the biomass is pre-milled to the required size range for the pulverised fuel boilers. Consequently, the immediate GHG savings in operational coal plants are highly cost-effective. The major drawback of this option, however, is that the share of cofiring fuel is limited and thus does not offer full decarbonisation. Unless it is equipped with carbon capture and storage (CCS) technology, this option can be seen only as a bridging technology towards fully renewable net zero emissions solutions.
More information is offered in this publication: The status of large scale biomass firing – the milling and combustion of biomass materials in large pulverised coal boilers
Waste-to-energy plants
Waste used as a feedstock for waste-to-energy plants is highly heterogenous and often contaminated. The feedstock can consist of residual waste streams from municipal solid waste (MSW), commercial and industrial (C&I) waste, and construction and demolition (C&D) waste. This calls for the use of robust technologies and extensive emission mitigation measures, leading to waste-to-energy facilities being associated with relatively high costs.
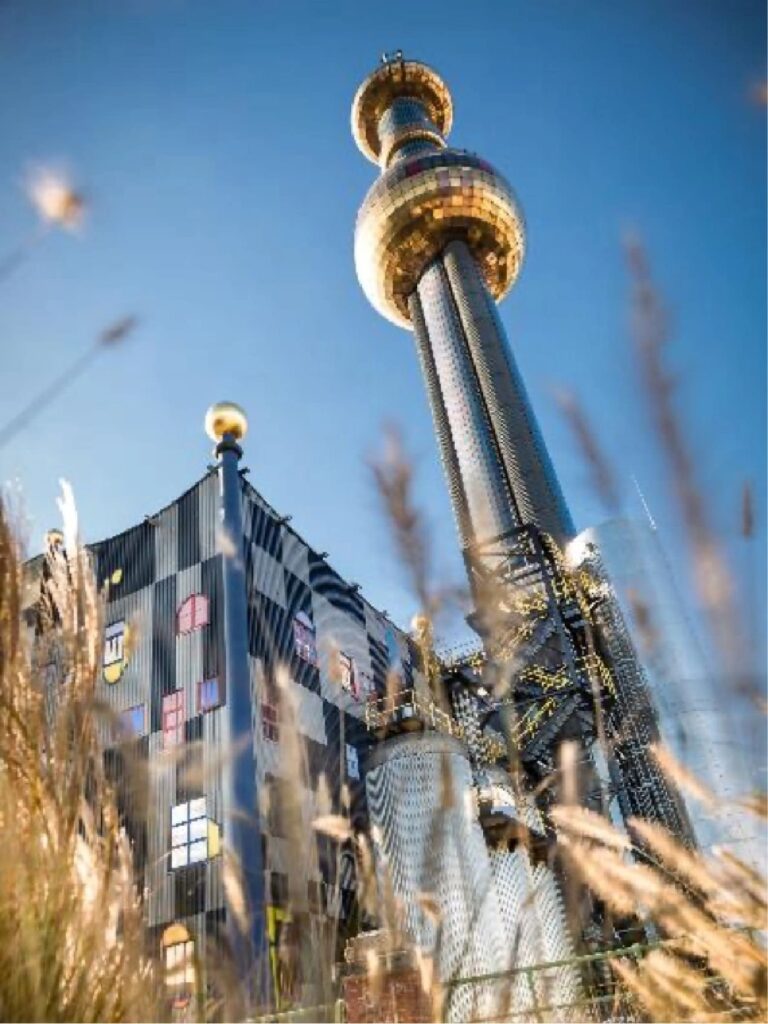
Different technologies are available, but the market to date has been dominated by incineration- based solutions that primarily apply grate-fired and fluidised bed boilers. There is currently a growing interest in alternative waste-to-energy technologies like gasification.
Waste-to-energy plants should not be seen as universal solutions for a malfunctioning waste- management system, but as part of an integrated approach to creating sustainable waste- management and energy systems.
Environmental effects
Greenhouse gas emissions
The combination of large-scale biomass power, CHP, or industrial applications with carbon capture and storage (CCS) is attractive in terms of achieving net-negative GHG emissions, but the current state of the art still falls well short of such solutions. Newly built plants focus on innovative technologies like chemical looping combustion, which features higher CO2 concentrations in the flue gas and thereby reduces sequestration costs. When it comes to the retrofitting of existing bioelectricity or biomass-based CHP technologies with CCS, the preferred alternative is clearly post-combustion separation of CO2, as this is currently commercially available.
The IEA Bioenergy project “Deployment of Bio- CS/CCU Value Chains” compiles case studies that provide deeper insights into the key aspects at stake for companies setting up value chains for capture, transportation, and sequestration or utilisation of biogenic CO2.
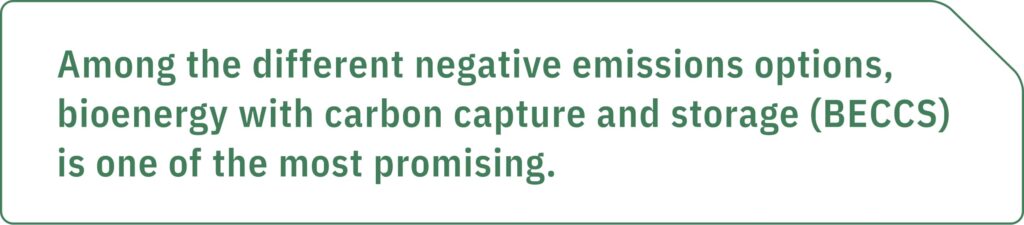
Emission of air pollutants
Biomass combustion will play an important role in our future sustainable energy system. To ensure low emissions of air pollutants, it is essential to support the implementation of state-of-the-art combustion technology and ensure appropriate operation. A powerful tool in this context is the international standardisation of product- and product- testing requirements accompanied by financial support schemes for high-quality technologies on the one hand and stringent emission legislation on the other. (Reference: Aerosols from Biomass Combustion – Technical report).
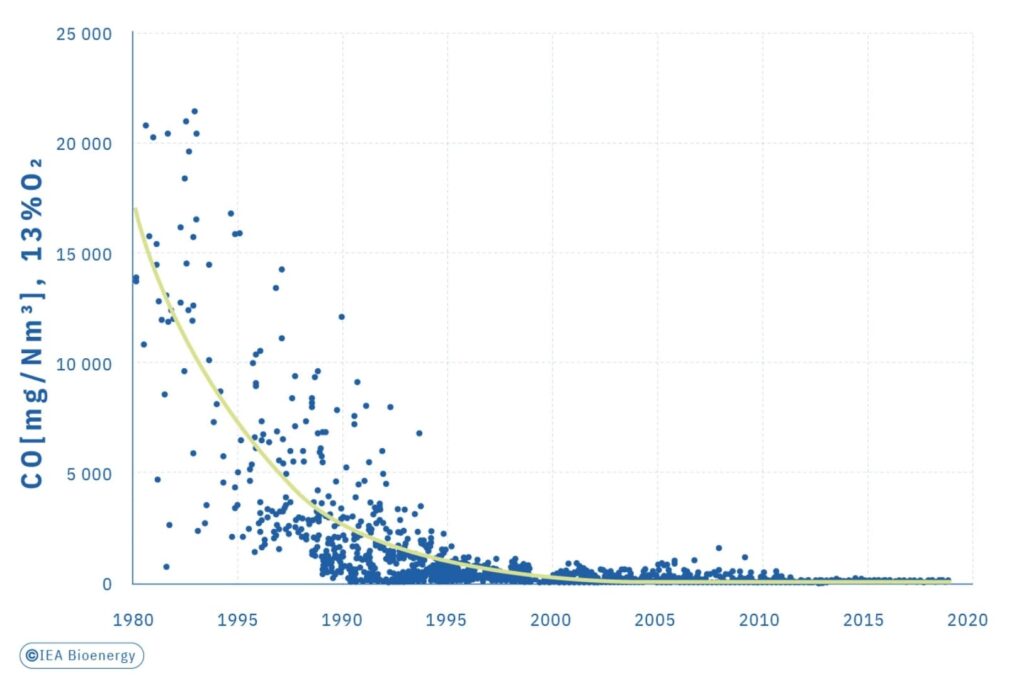
Costs
The profitability of a biomass combustion system is different for heat-only, power-only, and CHP systems. For residential heating applications, biomass combustion systems are often economically competitive under a variety of regulatory framework conditions. Larger-scale heating plants, such as those providing process heat applications in industry, usually require supportive economic environments with, for instance, carbon pricing or taxation systems in place, to make them competitive against fossil alternatives. In general, the economics of biomass combustion systems improve with higher heat demand and higher full-load hours.
For power and CHP applications, supportive regulatory framework conditions are essential. Further major influencing factors are biomass fuel costs and electricity market prices. Depending on electricity prices, biomass combustion-based power is often economically feasible only if financial supporting schemes such as feed-in tariffs or contracts for difference (CFDs) are available.
However, this is also the case for other renewable sources, as a recent study by Fraunhofer ISE concludes for the case of Germany. Levelised costs of electricity (LCOE) from biomass combustion systems were calculated at 7–15 €cent/kWh which, although higher than the average wholesale electricity prices of the past years, falls into a similar range to most of the other technologies investigated. Only utility-scale photovoltaics (PV) and on-shore wind power plants tend to reach lower LCOE, whereas coal and gas power are found at the upper end of the cost range of 20€cent/ kWh and above. For fossil fuel systems, moderate emission certificate prices (within an Emission Trading System) of 32–36€/t CO2eq were assumed.
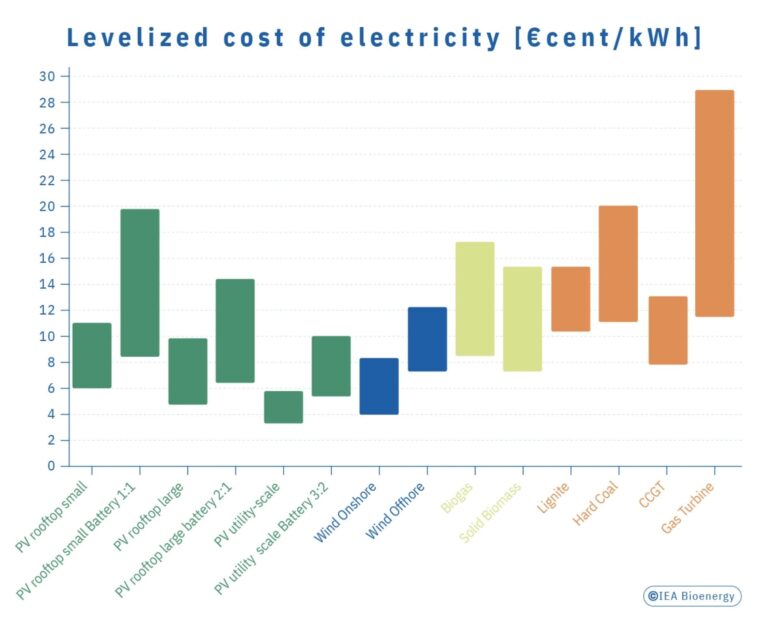
On a global scale, for most of the projects reviewed by the International Renewable Energy Agency (IRENA), LCOE is in the range of 0.05–0.15 USD /kWh, which is in line with the German case outlined above.
It is important to mention that all conclusions regarding the economy of biomass combustion systems are based on historic data. The extreme volatility of energy prices, particularly the steep increase in electricity and natural gas prices observed in the second half of 2021, is not considered in any of the studies mentioned above. If this turns out to be a long-lasting trend rather than a short-term market distortion, it will affect the (renewable) energy business.
Current research gaps and opportunities
Even if biomass combustion systems in all power ranges can be considered as mature technologies, significant research and development efforts are ongoing and the transformation of the energy system brings new challenges and opportunities that need to be addressed:
- Emission reductions still remain important in the case of small-scale appliances for residential heating, which typically come without secondary pollution abatement equipment, and also for medium- and large-scale combustion plants. Cost-effective solutions for reducing emissions of particulate matter and organic compounds in small-scale heating systems can expect good market opportunities, given that the sales figures for residential stoves and boilers are huge and emission regulation measures have been, and are being, tightened in many countries. For larger applications such as district heating systems, where complete combustion conditions widely avoid organic emissions, the reduction of NOx emissions is one of the major challenges.
- The transition away from fossil fuels in industry, in particular for high-temperature heat applications, is one of the most promising future market segments for biomass combustion technologies. The general trend towards greater electrification will also take place in many industries, but it is not well suited to all applications. Developing tailored sustainable energy solutions for providing heat to industrial processes is thus a promising new market segment for biomass combustion.
- Energy systems worldwide are under rapid development in order to comply with the climate change mitigation targets; they offer several unique opportunities for biomass combustion- based technologies. Increasing shares of wind and solar power are significantly improving the GHG balance of power-generation systems, but at the same time they are increasing the demand for grid balancing. Biomass combustion-based technologies offer the required high level of operational flexibility needed for grid balancing. At the same time, it is clear that additional efforts will be necessary to reduce GHG emissions in order to achieve the climate targets of the Paris Agreement. Biomass-based carbon capture and storage or utilisation concepts, where the carbon is sequestrated or synthesised into new products, can lead to net-negative GHG emission balances.
- Several technology manufacturers, following their vision of downsizing power plants to fit into every building, are developing robust and efficient small- and micro-scale CHP solutions. Most concepts are based on state-of-the-art wood pellets or wood-chip boilers which are equipped with different power units such as Stirling, micro-steam engines, or Organic Rankine Cycles (ORC). Due to the technological and economic challenges faced by such technologies, only a few products have entered the market. The idea of providing cost-effective CHP solutions for residential buildings, however, remains attractive, in particular as they nicely complement solar systems by providing energy during periods of low sunshine.
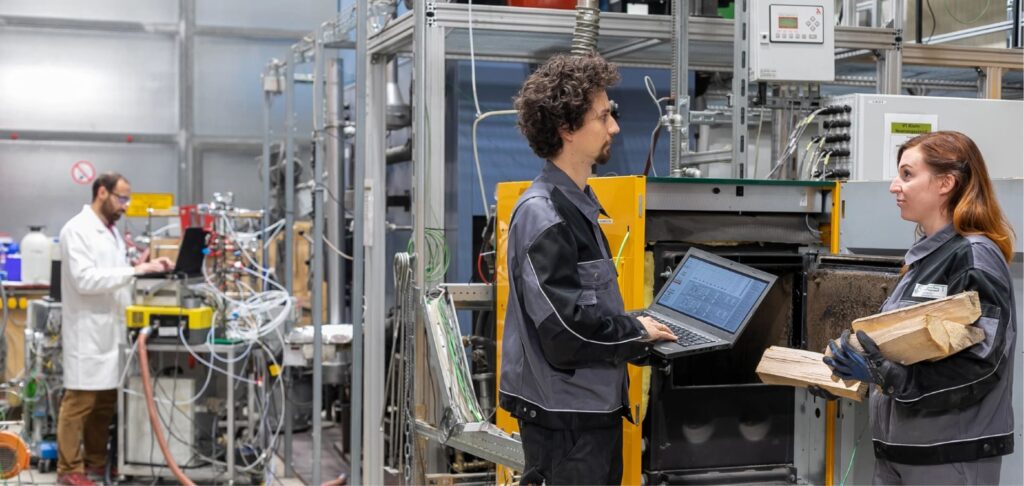
Powerful policy instruments
Many countries have implemented financial support schemes to stimulate the market penetration of more efficient modern biomass combustion systems. High capital expenditure (CAPEX) often poses a significant market barrier, especially for small-scale applications; several governments have thus implemented subsidy schemes that offer funding for the technology investment.
The actual implementation of such programmes differs from country to country, and even regionally or locally. In addition to such general financial incentives for renewable energy installations, specific programmes supporting the replacement of fossil fuel–based appliances have proven their effectiveness. Moreover, recently, such programmes have begun to include scope to replace old biomass-based technologies which can no longer be considered state-of- the-art in terms of efficiency and emissions.
For power applications, technology-specific feed-in tariffs or contracts for difference are the most common type of policy support. The procedures for defining the amount and the allocation of tariffs differ between countries. Today, most feed-in tariffs are constant over a defined period of time; however, new support systems tend towards CFDs that include compensation for the gap between the renewable power production cost and the actual electricity price.
Such dynamic systems are popular, as they reduce support costs when electricity market prices are higher. Once the shares of fluctuating renewable energy in the electricity system increase, the need for flexible and plannable production capacities may rise. In such a scenario, the value of electricity provided to the grid is likely to vary significantly according to the time (day/night, summer/winter), thus calling for dynamic financial support schemes.
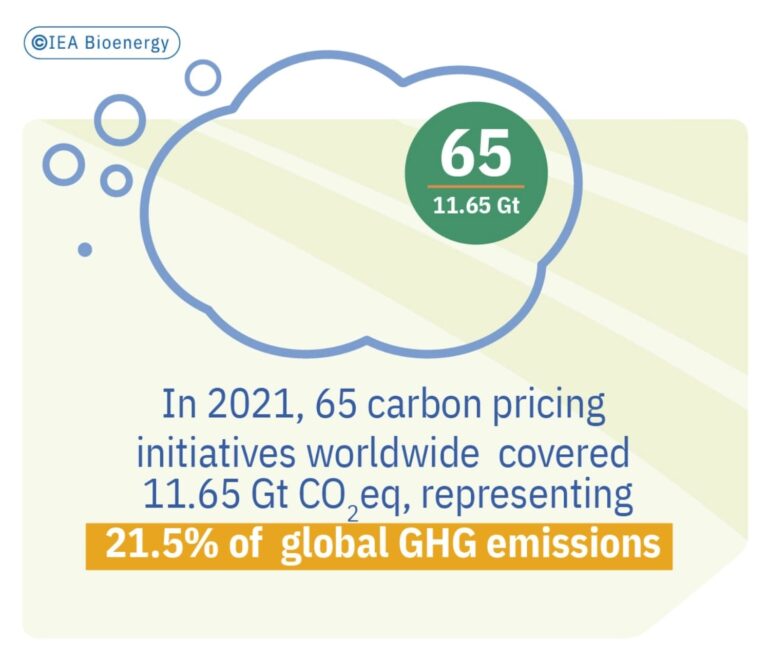
Completely different policy approaches aim to discourage people from using fossil fuel–based technologies. Measures such as carbon pricing or taxation systems, which impose taxes on fossil fuels and exempt or incentivise use of biomass fuels have already proven their effectiveness in Denmark. In 2021, 65 carbon pricing initiatives worldwide covered 11.65 Gt CO2eq, representing 21.5% of global GHG emissions. Sweden was the first country to introduce carbon pricing and has the highest carbon price in the world of almost 140 USD/t CO2eq; this has proven effective at driving decarbonisation.
Alternatively, the use of fossil fuels can also be prohibited directly or indirectly, as is currently the case for heating oil in residential applications (e.g., in Austria).
A recent report of Task 32 highlights the opportunities for bioenergy technologies to deliver heat in industry, and compares these with alternatives to decarbonisation such as CCS, electrification, and hydrogen. Specific policy recommendations are provided to accelerate their adoption.
- QM Biomass District Heating Plants LINK accessed 18/02/2022
- IEA Bioenergy Task 32 (2018) Bioenergy for heat – the Hot Cases, strategic study on renewable heat LINK accessed 18/02/2022
- IEA Bioenergy (2020) Bioenergy for High Temperature Heat in Industry – Case Study 1: Wood chips combustion for process steam in a potato processing industry LINK accessed 18/02/2022
- IEA Bioenergy (2020) Bioenergy for High Temperature Heat in Industry – Case Study 5: Combustion of wood chips and grain residues for process heat supply in the largest bakery in Switzerland (2021) LINK accessed 22/06/2022
- IEA Bioenergy Task 32 (2019) Best practice report on decentralized biomass fired CHP plants and status of biomass fired small- and micro scale CHP technologies.LINK
- IEA Bioenergy (2018) Biomass pre- treatment for bioenergy Case study 4: The steam explosion process technologyLINK accessed 22/06/2022
- IEA Bioenergy (2019) Fuel treatment of biomass residues in the supply chain for thermal conversion LINK accessed 18/02/2022
- IEA Bioenergy Task 32 (2016) The status of large scale biomass firing The milling and combustion of biomass materials in large pulverised coal boilers LINK accessed 22/06/2022
- European Commission (2015) Science and Policy Reports, Solid and gaseous bioenergy pathways: input values and GHG emissionsLINK accessed 18/02/2022
- IEA Bioenergy, Deployment of bio-CCS: case studiesLINK accessed 22/06/2022
- European Union First call for large-scale projects list of proposals pre-selected for a grantLINK accessed 18/02/2022
- IEA Bioenergy Task 32 (2017) Aerosols from Biomass CombustionLINK accessed 18/02/2022
- Kost C, Fraunhofer ISE (2021) Study: Levelized Cost of Electricity- Renewable Energy TechnologiesLINK accessed 18/02/2022
- IRENA (2021) Report International Renewable Energy Agency “Renewable Power Generation Costs in 2020”LINK accessed 18/02/2022
- IEA Bioenergy Task 32 (2019) Bioenergy The future role of Thermal Biomass Power in renewable energy systems- study of GermanyLINK
FURTHER READING
- IEA Bioenergy Task 40 (2020) Deployment of BECCS/U value chains – Technological pathways, policy options and business modelsLINK accessed 18/02/2022
- IEA Bioenergy Task 32 (2021) Webinar: Residential Wood Combustion – Towards Low Emission SystemsLINK accessed 22/06/2022
- IEA Bioenergy Task 32 (2018) Status of PM emission measurement methods and new developmentsLINK accessed 22/06/2022
- IEA Bioenergy Task 32 (2018) Advanced Test Methods for Firewood Stoves – Report on consequences of real-life operation on stove performanceLINK accessed 22/06/2022
- IEA, Report Global EV Outlook 2021 (2021)LINK accessed 18/02/2022
- IEA Bioenergy Task 32 (2019) Future of thermal biomass powerLINK